CRISPR: Cutting And Pasting The Genome - Blog - Reeve Foundation
It is now possible, with minimal technical skill and very little cost, to essentially edit and perhaps correct the human genome. The genome comprises the basic blueprint for all organs, tissues and cells in the body, and for all bodily functions, including those associated with disease or those damaged by trauma. Cut-and-paste gene editing technologies, including the newest one, CRISPR-Cas9, allow direct modification of the full genome.
This possibility of personalized, molecular surgery has been a giant bolt of energy in biology, sparking both optimism and concern. Yes, it will soon be used in humans. Editing or correcting a gene that encodes a damaged or missing protein may very well result in full correction of disease. But what about editing the mother code, in an embryo? Correcting a gene there will pass the edit on to future generations. For now, the eugenics approach has been very much discouraged by the gene-mod community. Designer mutations are inevitable; it’s only a matter of time. There’s a lot to work out first.
CRISPR, for sure, is a game changer. Potentially revolutionary. There are scientists looking to use this tool to splice out HIV, various types cancer, hepatitis B, muscular dystrophy, or even autism. On the farm and ranch side, researchers are looking at ways to modify crops and livestock; they’ve made tiny pigs, disease-resistant rice and vitamin-enriched oranges.
CRISPR has entered the realm of spinal cord injury research, as we will discuss below with Sam Pfaff, Ph.D., whose lab at the Salk Institute is one of the seven in the Reeve International Consortium on Spinal Cord Injury. There are scientists working with gene editing with an eye on clinical applications in SCI, says Pfaff, including ways to turn on axon growth, or to amplify or mute various growth factors. First a bit of background.
CRISPR stands for Clustered Regularly Interspaced Short Palindromic Repeats; yes, they read the same backwards or forwards (a man, a plan, a canal, Panama). These code patterns occur in the genome of certain bacteria, from which the new editing system, CRISPR-Cas-9, was discovered. (Bacteria, it seems, have adapted a sort of immune system; they fight off repeated virus attacks using precise DNA targeting).
Cas9 is a CRISPR-associated enzyme that has been likened to “molecular scissors” to cut and edit DNA in any cell. A guide RNA can be custom-synthesized in the lab to match -- with a remarkable GPS-type accuracy -- the specific gene target. The RNA directs the Cas9 scissors to an exact site on a cell’s DNA. Snip and replace. Paul Knoepfler, a stem cell researcher at UC Davis, thinks of CRISPR as a molecular Swiss army knife: part genome scanner (like a magnifying glass), part scissors, and part pencil to overwrite genetic code.
In 2012, Emmanuelle Charpentier, a German microbiologist and Jennifer Doudna, from the University of California, Berkeley, used a single RNA with Cas9 to slice DNA sequences in test tubes. A few months later, Feng Zhang, a biologist at the Broad Institute at MIT, and George Church, a Harvard geneticist, separately reported that the CRISPR/Cas9 system could edit the cells of humans. It’s a sideshow but a good one: Cal vs. MIT for the intellectual property rights. Zhang has the patent for now but Berkeley has challenged and will get another shot at it. Here’s the latest patent update from Nature.
Sam Pfaff
The Pfaff lab is not involved in developing the gene editing technology but has been successful in taking advantage of it as a tool to study the genetics and function.
“We use CRISPR to make mutations in the mouse genome,” says Pfaff. “The mouse has been an incredibly important biological tool for genetics for a very long time. One of the big revolutions in mouse biology or mouse genetics has been in gene targeting, using homologous recombination techniques in embryonic stem cells; this is used as a way to knock things into loci or to create mutations to study the function of genes. As a tool, homologous recombination is not extraordinarily efficient. It takes a degree of brute force, transecting the mouse embryonic cell, selecting out clones and analyzing hundreds of them using rather tedious kinds of techniques such as southern blocks. Once the clone is identified it has to be injected into mouse embryos; then you have to wait to see if the ES cells contribute to the germ line [to make the intended knock-out mouse line].”
It’s a tedious process, Pfaff says. “It’s not uncommon that for a new mouse line to take a full year to develop. A year is a good amount of time in the life of a post doctoral fellow, and is a considerable cost to the laboratory. But hundreds of genes have been targeted that way; until a couple of years ago, that was the state of the art.”
CRISPR dramatically streamlines the process. Says Pfaff, “We can now synthesize the genetic sequences needed for base pairing with little or no need for cloning. This is indeed a game changer. Rather than make a mutation in an embryonic stem cell, which we then interject into mouse embryos, we can go right to the fertilized oocyte. We skip the embryonic stem cells altogether -- the progeny are born three weeks later. We can then screen the animals directly: Do they have the mutation or don’t they.” He says entirely new strategies for performing gene screens are now taking place. “Literally, libraries are being made to target every gene in the genome.”
Pfaff notes that CRISPR constructs are relatively simple to make, and quite accurate. “Now,” he says, “the efficiencies for CRISPR are so high, we, and others, are contemplating this in a clinical context; it is not beyond the realm of possibility that we could, for example, go in to edit the genes related to a metabolic disorder or to modify a tumor cell.”
So why make mouse mutations? “One of the more rigorous scientific ways that we can test the function of a gene,” says Pfaff, “is to ask the question, what are the biological consequences of preventing that gene to function? A mutation therefore eliminates the function of a specific gene. If we eliminate specific genes in the spinal cord, we can ask, for example, do neurons then degenerate, or do they grow better because a gene we targeted represses neuron growth?”
A major part of Pfaff’s research is understanding the underlying circuitry of the spinal cord. CRISPR is an important new tool. “With the ability to tag specific cell types by virtue of their unique gene expression,” says Pfaff, “we can better understand how the system relays sensory information, or how it regulates motor commands. We can purify very specific cell types and look at their molecular composition; this allows us to do electrophysiology on particular neuron types, so we can label them and monitor them. We are able to track the cell in real time and ask, under various conditions, what happens to survival of the cell, what is its physical, architecture, what is its connectivity.”
Just how easy is CRISPR? “Even someone with limited experience in molecular biology ought to be able to work with the CRISPR platform,” says Pfaff. “The protocol is relatively simple. Some of the older methods [for gene modification], it’s almost as if they are an art form; it takes someone who’s been doing it for years to make it work. With CRISPR, it’s not so specific.”
Because one can activate or repress any gene and therefore any cellular function in the body, it’s not hard to imagine others in the SCI research field grasping on to CRISPR. Pfaff says the technology is being widely experimented with.
“Colleagues in many areas of study in the spinal cord are quite interested in it. If you know, for example, about a repressive axon guidance molecule, in a myelin associated glycoprotein, for example, or perhaps in a developmental program, it may be possible to activate genes to spur growth of new nerve cells. It might be possible to create a better environment for cell survival, or to restore neuronal function by restoring connections, promoting growth of more cells that might replace those damaged by injury. In my lab we are using CRISPR in more of a research context. It is not at all far fetched to envision, down the road, that CRISPR could be a very useful tool in our arsenal of gene therapies."
“A year ago it was trendy to say I’m using CRISPR. Now, it’s to the point that if you’re not using it, why are you so behind the times?”
For more on CRISPR, see this nicely done animation from MIT above.
Great overview article from a recent New Yorker.
See also the New York Times.
The journal Nature produced a very detailed special edition, CRISPR: the good, the bad and the unknown.
Join Our Movement
What started as an idea has become a national movement. With your support, we can influence policy and inspire lasting change.
Become an Advocate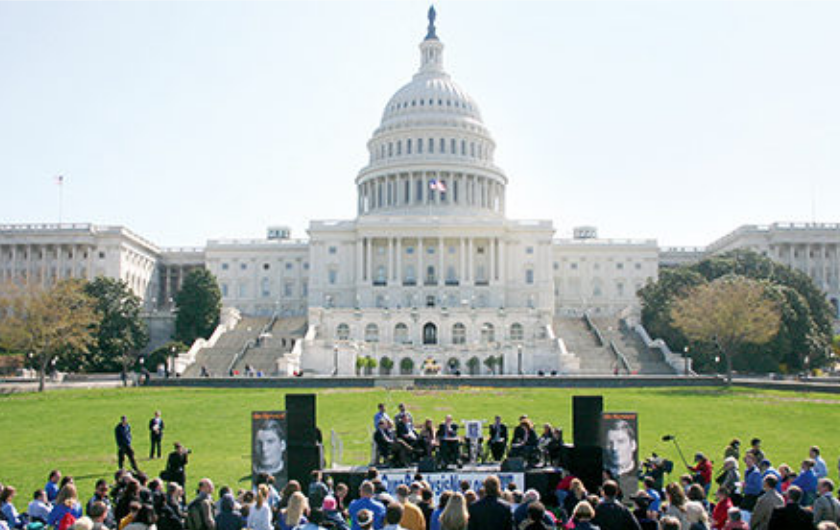